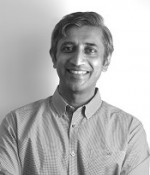
Hiten Madhani, MD, PhD
Our laboratory is located on the third floor Genentech Hall at the UCSF Mission Bay Campus. We investigate the biology of Cryptococcus neoformans, the most common cause of fungal meningitis. As an opportunistic pathogen, this encapsulated yeast is the major fungal driver of mortality in HIV/AIDS, where it is estimated to cause up to 1/3 of deaths in this patient population, thereby exceeding the worldwide death toll from breast cancer. As a model organism, C. neoformans is highly tractable, offering haploid genetics and facile gene targetting. It is thus well-suited for addressing important biological questions using a range of powerful cutting-edge approaches. Our current work is divided into translational and basic science areas:
1. Fungal pathogenesis
Infections represent quintessential conflicts between microbes and hosts and have had a major impact on human history. Fungal infections are an especially difficult class of infections to treat, resulting in high morbidity and mortality. It is estimated that 50% of AIDS-related deaths are due to fungal infections. Chief among these is fungal meningitis caused by C. neoformans, which is responsible for 500,000 annual deaths, greater than the annual worldwide toll from breast cancer. We seek to identify and understand the specializations that endow C. neoformans with the ability produce a lethal infection in mammals.
In our early studies, we sought to investigate virulence factors by targeted gene deletion. Unfortunately, the genome sequence was unannotated, transformation was cumbersome (requiring biolistics --“a gene gun”), and homologous recombination was inefficient even with kilobases of targeting homology. Nonetheless, by developing homology-based gene annotation and optimizing biolistic targeting, we succeeded in constructing 1200 targeted mutations in our initial effort. We screened these mutants in mice and in vitro to identify several dozen genes required for success in the host but not for growth in vitro under a variety of conditions or for the production of known virulence factors such the polysaccharide capsule (Liu et al., 2008). Our ability to find numerous novel virulence genes in a nonsaturating genetic screen implied that much remained to be discovered. Early on, we identified adaptation to hypoxia as a key pathogenicity determinant that is mediated by a pathway homologous to the human SREBP sterol homeostasis pathway (Chun et al., 2007). Subsequent work showed that the pathway is conserved in another lethal fungal pathogen, Aspergillus fumigatis, where it is also required for hypoxic adaptation and virulence. Taking advantage of refined gene models, we are well on the way towards constructing a complete nonessential gene deletion collection in C. neoformans.
Host-pathogen conflict: phagocytosis and its evasion
C. neoformans effectively evades phagocytosis by macrophages, a central cell type of the innate immune system. Progress towards understanding why this is the case came from our identification of a C. neoformans transcription factor whose inactivation results in strongly increased susceptibility to phagocytosis by macrophages and a loss of virulence (Chun et al., 2011). Importantly, neither defects in the polysaccharide capsule nor exposure of known fungal molecular patterns is responsible for the phenotype, again implying novel mechanisms. We identified the direct targets of the transcription factor and found among these a family of highly expressed, signal sequence-bearing proteins harboring a double-psi beta barrel of unknown function. We have clear evidence that at least one member of this family is required for phagocytosis inhibition (Chun et al., 2011). We will continue to investigate this protein family to elucidate their precise molecular roles virulence and the inhibition of phagocytosis. Specifically, we will investigate the possibility is that the pathogen produces a ligand for receptors on phagocytes that recognize “don’t eat me” signals on normal cells that prevent self-phagocytosis. These receptors are rapidly evolving, suggesting conflict with pathogens.
Cell-cell communication and virulence
Quorum sensing systems are instrumental regulators of population behavior in bacteria, which control community activities ranging from bioluminescence to virulence. However, whether eukaryotes possess analogous systems is unclear. We have identified a gene encoding a secreted peptide, Qsp1, as a direct target of three transcription factors that we had discovered in earlier studies to be required for virulence (Chun et al., 2011; Liu et al., 2008). We found that deletion of this gene produces cells that grow normally but are highly attenuated for virulence in the animal. The mutant also exhibits changes in surface properties evident as increased adhesiveness and altered colony morphology. Remarkably, wild-type cells or the purified peptide can complement these in vitro defects in trans, indicative of cell-cell signaling. Investigations of the role of cell-cell communication in pathogenesis are a major current focus.
2. Genome Defense
The emergence of the earliest life forms was likely quickly followed by the appearance of the first selfish nucleic acid parasites. Whatever their form, these could have extinguished early life (via genome damage or the exhaustion of cellular resources) were it not for the evolution of cellular countermeasures. Transposable elements are a ubiquitous class of endogenous parasites that hitchhike on the host genome. Because they violate Mendel’s Laws, sexual reproduction allows transposons to spread through populations despite a negative impact on host fitness. Clustered repeats, sometimes referred to as satellites, are also a major driver of genome expansion and instability. The risk for species extinction has been linked to genome size increases, suggesting a profound impact of selfish nucleic acids on evolution.
The intrinsically deleterious nature of genomic parasites, together with their ability to out-replicate the rest of the genome, likely drove the evolution of a rich set of defense mechanisms. The primary mechanisms by which eukaryotic cells silence transposons and other repeated sequences are small silencing RNAs and chromatin modifications. Whereas the core biochemistry of both mechanisms is well-developed, the central question remains unanswered: how do cells distinguish “normal” from threatening nucleic acid sequences? Without the ability to distinguish “good” from “bad” nucleic acids, any genome defense scheme would fail. Our work focuses on this question. In mechanistic terms, it translates into “what determines the templates for small RNA synthesis and what defines where silent chromatin marks are placed”? The answers have been full of interesting surprises.
Stalled spliceosomes are a signal for RNAi-dependent genome defense
We have discovered a fundamentally new function for introns and the spliceosome in RNAi-dependent genome defense (Dumesic et al., 2013). Unlike S. cerevisiae and S. pombe, C. neoformans uses RNAi to suppress the movement of transposable elements. We sequenced the small RNA repertoire of C. neoformans and discovered that siRNAs are surprisingly generated from both intronic and exonic sequences. We characterized a nuclear RNA-dependent RNA polymerase complex, SCANR, required for siRNA biogenesis, and found that it associates with an SR-like protein that itself is a component of the spliceosome. We also noticed that pre-mRNAs that template siRNA synthesis display suboptimal splicing features, particularly in terms of length. This led us to hypothesize that the stalling of spliceosomes is a necessary signal for targeting pre-mRNAs to SCANR for double-stranded RNA synthesis. This model predicted that pre-mRNAs targeted for RNAi should be stalled on the spliceosome and that introns would be required for siRNA production. Furthermore, the model predicted that a normally poor RNAi candidate could be converted into a strong one if stalled splicing of the transcript could be engineered. Each of these predictions was experimentally verified. Moreover, we identified the lariat debranchase as an essential siRNA biogenesis factor, suggesting that the lariat-intermediate product of the first catalytic step of splicing is a precursor to dsRNA.
Our results address a profound mystery in eukaryotic biology: why genes-in-pieces? More generally, our studies promote a paradigm in which the efficiency of gene expression is used as a metric to distinguish which nucleic acids need to be silenced (Dumesic and Madhani, 2014). Indeed, many aspects of eukaryotic gene expression ranging from chromatin to mRNA modifications to mRNA quality control mechanisms may have been selected for because of the need to protect cells from parasitic nucleic acids (Madhani, 2013a).
Understanding how stalled spliceosomes lead to the production of siRNAs is a major current focus. We plan to employ a combination of genetic and biochemical approaches to understand how stalled spliceosomes engage siRNA synthesis. Our pursuit of a mechanism is inspired by strong hints that the principles will turn out to be conserved in animals and plants (Dumesic and Madhani, 2013). We also plan to investigate if and how transposons escape spliceosomal surveillance. Finally, we are applying forward genetics to investigate how transposable elements are tightly repressed by small RNAs.
HP1-dependent chromatin silencing of repeats
Since the electrifying discovery of the first chromatin-modifying enzyme in the mid-1990s and its homology to a yeast protein required for transcription (Brownell et al., 1996), the field of chromatin biology has grown rapidly. Early investigations of chromatin modification in my laboratory demonstrated that the histone variant H2AZ functions in euchromatin to prevent the ectopic spread of heterochromatin in S. cerevisiae from repetitive regions (Meneghini et al., 2003). This finding changed our view of chromatin because it indicated that euchromatin is not a default state on which heterochromatin was assembled but rather that both types of chromatin contain mutually antagonistic modifications. In collaboration with Jasper Rine (UC Berkeley), we identified the Swr1 complex required for H2AZ deposition genomewide (Kobor et al., 2004). We also discovered the ubiquitin ligase, Bre1, responsible for euchromatin histone H2B monoubiquitylation (Hwang et al., 2003). We next discovered that H2AZ is deposited at nucleosomes that flank a nucleosome-free region (NFR) that characterizes eukaryotic promoters (Raisner et al., 2005). Subsequently, we defined widely acting DNA binding proteins and a chromatin-remodeling enzyme that act in a pathway to produce this promoter nucleosome pattern (Hartley and Madhani, 2009). We also found that, in vivo, nucleosome-free regions are upstream of H2AZ deposition, suggesting that the NFRs trigger H2AZ deposition. These early studies revealed mechanisms that instruct euchromatic chromatin organization.
Because of our interest in repeat sequence and transposon silencing, we shifted our studies fromS. cerevisiae to S. pombe when it became clear that histone H3 lysine 9 methylation (H3K9Me) is a conserved mark of heterochromatin in S. pombe and many other species but that this mark was lost during the evolution of S. cerevisiae. In S. pombe, H3K9Me recruits HP1 proteins to pericentromeric and other repeat sequences. We used single nucleosome mapping to show that the silencing machinery triggers elimination of nucleosome free regions thereby limiting access of transcriptional regulator proteins to DNA (Garcia et al., 2010). In collaboration with Geeta Narlikar (UCSF), we have investigated how HP1 bridges nucleosomes (Canzio et al., 2011; Canzio et al., 2013) and functions with the Clr4 histone methyltransferase to accomplish silencing (Al-Sady et al., 2013). We have also studied how boundaries are formed between heterochromatin and euchromatin, in the process discovering a Cul4-type ubiquitin ligase that sculpts the heterochromatic landscape via regional degradation of an anti-silencing factor (Braun et al., 2011).
Despite great progress in understanding the fundamental biochemistry of heterochromatin assembly, the question of what determines why repeats and transposons are selected for heterochromatin assembly remains unsolved. The finding that RNAi plays a role in heterochromatin assembly in fission yeast over ten years ago was an important discovery. Curiously, while RNAi plays an important role in establishing H3K9Me in S. pombe, it is largely dispensable for its subsequent inheritance at endogenous sequences. Likewise, siRNAs are insufficient to trigger stable heterochromatin assembly. These observations suggested the existence of RNAi-independent mechanisms for propagating RNAi-triggered histone methylation. A key breakthrough was our identification of a sequence-specific ncRNA-binding protein, Seb1, that mediates this RNAi-independent pathway (Marina et al., 2013). We demonstrated that this protein acts via recruitment of a deacetylase/remodeling complex (Marina et al., 2013). We are investigating the concept that heterochromatin can be epigenetically inherited independently of an initial RNAi trigger, but only if licensed by specific ncRNA signals.
Polycomb in yeast
Polycomb repressors mediate the formation of facultative heterochromatin and play extraordinarily important roles in animal and plant development and in many human cancers. They also function to silence repeats. Dissecting how these repressors work would clearly benefit from a tractable unicellular system. However, Polycomb was lost in almost all fungal lineages including those that gave rise to S. cerevisiae and S. pombe.
We have discovered that orthologs of components of the Polycomb Repressive Complex 2 (PRC2) are retained in Cryptococcus and, furthermore, they form a five-protein complex that strongly resembles the human complex. This complex deposits the H3K27Me3 mark at repetitive subtelomeric regions thereby silencing genes in these regions, which are enriched for nutrient transport and catabolism genes as well transposable elements.
We have obtained remarkable results with one subunit of the C. neoformans PRC2 complex, a Polycomb-like chromodomain-containing protein. The chromodomain of this protein directly binds to the H3K27Me product, and our analysis revealed that mutational disruption of mark recognition results in a new genome-wide pattern of H3K27Me that, strikingly, coincides with the pattern of H3K9Me. Introduction of an additional mutation that removes all H3K9Me results in the concomitant loss of all H3K27Me. Thus, the PRC2 complex in Cryptococcus posseses an intrinsic “latent promiscuity” that attracts it to H3K9Me heterochromatin. This tendency is normally masked by the ability of the complex to recognize its enzymatic product.
We will continue to investigate this system to understand how the two types of repressive chromatin (constitutive vs. facultative) are separated, the normal biological role of PRC2 in this organism and the mechanisms that recruit PRC2 to repetitive subtelomeric regions.
8/30/2021 - DEI Champions Training
Websites
- Madhani Lab
- UCSF Profile
- LinkedIn Profile
- TETRAD
- Biophysics Profile
- Bioinformatics Profile
- California Institute for Quantitative Biosciences
- Helen Diller Family Comprehensive Cancer Center