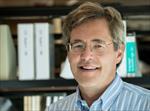
Bruce Conklin, MD
Therapeutic Approaches to Genetic Disease
Genome Engineering
The late Richard Feynman once said, “What I cannot create, I do not understand.” Human genetics began as observational science, but newly developed genome-engineering tools now allow us to directly test the cellular consequences of discrete genetic changes. We have developed efficient methods to edit one residue at a time in living human induced pluripotent stem (iPS) cells, resulting in “isogenic” lines of iPS cells. These isogenic lines form models that are now yielding phenotypes that are helping to explain the molecular basis of several human diseases. We are constructing collections of these isogenic lines that carry a range of disease mutations, from the most severe (rare) to the moderate (common) forms of cardiomyopathy.
Precise Genome Editing for Human Therapy
The rapid development of genome engineering, such as with the CRISPR system, now allows us to contemplate using these tools for human therapy in selective tissues. Using genome editing to “fix” a disease gene has great promise, because it could permanently correct the disease. However, tremendous challenges remain to ensure that only a specific part of the genome is edited, without causing “off-target” DNA damage that could lead to cancer. We focused on developing therapeutic editing for diseases of the heart and retina, because each tissue has unique challenges and opportunities. The heart has many newly described gene mutations that result in lethal heart failure (cardiomyopathy). Genome engineering can be used to cure cardiomyopathy mutations and validate targets for drug therapy; however, current engineering methods will need to be greatly enhanced before it can be used in vivo in the heart. In contrast, the retina has the advantage of a limited number of cells that can be directly targeted for therapy. These properties could potentially cure blindness caused by many gene mutations that currently have no therapy. We are developing proof-of-concept therapeutic editing methods in the retina that can be expanded to other tissues, such as the heart.
Human Cardiac Disease Models
We use iPS cells to model the genetic causes of human cardiomyopathy. Surprisingly, little is known about many genes associated with heart failure, from cardiomyopathy to abnormal heart rhythm, that results in “sudden death.” The heart provides an ideal system to determine the molecular basis of gene associations in human genetics. Until recently, modeling human gene variants in human cardiac tissue has been impossible. Human iPS cells now allow us to produce cardiovascular tissues that are identical, except for a single gene that has been altered by genome engineering. Our efforts have already been used to uncover multiple disease phenotypes and targets for drug therapy.
CRISPR-Based Screens in Human Cardiac Disease Models
Recently, we developed CRISPR-inhibition (CRISPRi) cell lines for highthroughput gene inactivation of thousands of different genes. CRISPRi screens can be used to identify the molecular basis of development, so that we can construct more mature human tissues and improved disease models. These studies will also allow us to identify drug targets that could be used for treating cardiomyopathy and other major diseases. We are currently focused on finding new pathways to enhance cardiac regeneration. In the future, other versions of the CRISPR system could be used to activate genes and control the epigenetic state of the genome.
Future Directions
We are developing new genome engineering methods in human iPS cells to identify therapeutic targets in cardiac disease. We are using these same tools to develop strategies for therapeutic genome editing. The combination of human iPS cells and genome editing provide unprecedented opportunities to explore new areas of biology and discover new therapies for disease.
Selected Recent Publications
1. Mandegar MA, Huebsch N, Frolov E, SHin E, Weissman JS, Qi LS, So P-L, Conklin BR. (2016) CRISPR interference efficiently induces gene knockdown and models disease in iPSCs. Cell Stem Cell. 18 1-13, April 7, 2016
2. Huebsch N et al. (2015) Automated video-based analysis of contractility and calcium flux in human-induced pluripotent stem cell-derived cardiomyocytes cultured over different spatial scales. Tissue Eng. Part C Methods 21:467.
3. Ma Z et al. (2015) Self-organizing human cardiac microchambers mediated by geometric confinement. Nat. Commun. 6:7413.
4. Miyaoka Y et al. (2014) Isolation of single-base genome-edited human iPS cells without antibiotic selection. Nat. Methods 11:291.
5. Spencer CI et al. (2014) Calcium transients closely reflect prolonged action potentials in iPSC models of inherited cardiac arrhythmia. Stem Cell Reports 3:269.
9/2021 - Neuroscience Graduate Program - Disabilities Awareness Training
10/2021 - CCMBM - "Welcome to the Lab" Letter Workshop